In this case study, we hear from Amalie Stokholm (based at the School of Physics and Astronomy), who has been using stars to gain insights into the history of our Galaxy using the high-performance cluster BlueBEAR and the Research Data Store, both housed at the University of Birmingham.
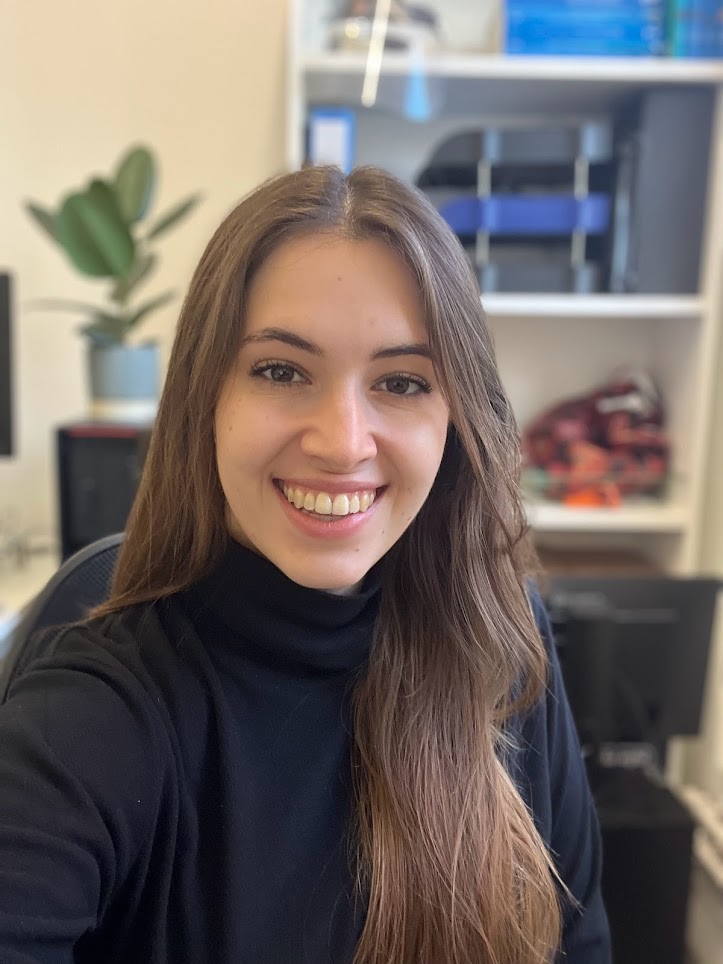
My name is Amalie Stokholm. I am a research fellow based at the School of Physics and Astronomy in the Sun, Stars, and Exoplanet group. I study the stars in our home galaxy, the Milky Way, I aim to contextualise the intrinsic patterns in their properties within the broader galactic context, using stars to gain insights into the history of our Galaxy. To achieve this, I utilise the storage and data processing capabilities of BEAR to simulate and model thousands of stars systematically. This approach allows me to accurately determine key parameters such as masses, chemical compositions, and ages of real stars in the Milky Way.
Stars are fascinating because they are formed throughout the Galaxy’s lifetime, carrying imprints of their birth conditions and their subsequent history. This means they hold clues about how our Galaxy has evolved and developed over time. We can use the stars as time capsules to reconstruct the timeline of what happened when and at what rates. The ultimate goal of my research is to pinpoint when key events occurred in the Milky Way’s history and understand how a galaxy evolves from the Big Bang to the present day.
The stars I study are quite similar to our Sun in many aspects, but they are more advanced in their evolution than our Sun. Currently, our Sun is in a stable phase of its life, shining due to fusion processes in its core. There it converts the lightest element in the Periodic Table, hydrogen, into the second-lightest element, helium. This fusion process generates a significant amount of light and energy, some of which reaches us here on Earth.
I have tackled similar tasks before before but never on the scale required by my current research project, nor on a computer cluster infrastructure as sophisticated as the one we have here
Our Sun still has plenty of fuel to sustain this heartbeat fusion process for billions of years. However, the stars I study are much further along in their lifespans. They have exhausted the fuel in their cores and can no longer shine by converting hydrogen into helium – the core of these stars is now essentially a sphere of helium. Gravity takes over, causing the core to contract and heat up. With these higher core temperatures, energy can be generated by converting hydrogen to helium in a shell surrounding the core. Additionally, the outer layers of the star expand, making the star grow in size up to tens of thousands or even millions of times larger. As the outer layers spread over a much larger area, the star appears more orange or red in the night sky.
Why do I care about these kinds of stars? Firstly, they are quite common, so the patterns I discover in them can reflect the general trends of the stellar populations. Secondly, because these stars are much larger than our Sun, they shine brighter and can be observed and measured over considerable distances. This is crucial for my research as I aim to learn not only about our solar neighbourhood but about the entire galaxy. Understanding how stars farther away differ from our Sun and its closest neighbours is essential for revealing the broader characteristics and evolution of the Milky Way.
They [BEAR] also helped finding extra storage space so this computation was possible in the first place.
I aim to translate the observable information about stars, such as their brightness measured across different sets of wavelengths or measurements of different chemical elements in their atmospheres, into more difficult-to-measure-directly properties, such as the stellar mass, the radii, or the age of the star.
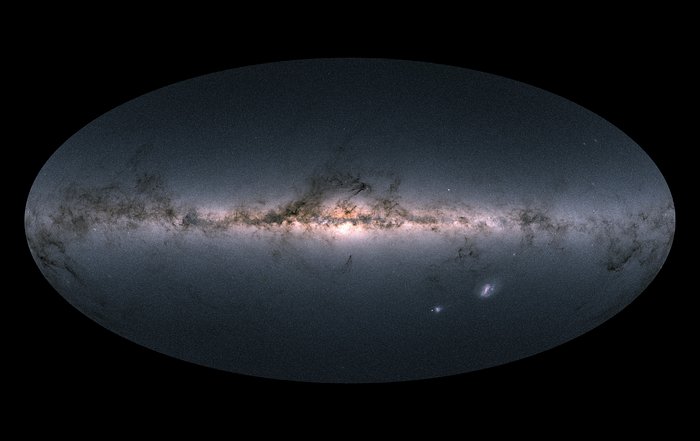
This is not a simple task. The current theory of stellar structure and evolution is incomplete, so we need to make reasonable assumptions and address several numerical challenges. There are multiple degeneracies among interesting properties, making it crucial to handle the uncertainties and correlations between measurements in a consistent and realistic manner. Failing to do so could easily lead to incorrect conclusions from the data.
Whenever people ask me about my experience at the University of Birmingham, I always emphasise BEAR.
I tackle this task by using a Bayesian framework, which enables me to properly account for the measured uncertainties. To apply my systematic approach, I model thousands of stars, varying numerous conditions and properties. Additionally, I let these models evolve over time to incorporate the temporal dimension necessary for inferring the ages of the stars.
Our current approach to tacking this issue is quite demanding in terms of storage space. I need frequent snapshots of the structure and conditions of my simulated stars as their properties change over their lifetimes. While one summary file of this output doesn’t take up much space, the computations themselves require gigabytes of space. When multiplied by the resolution I need, this adds up to multiple terabytes.
I have never encountered a computer cluster, storage system, and infrastructure that operate as smoothly and reliably as they do here.
I have tackled similar tasks before before but never on the scale required by my current research project, nor on a computer cluster infrastructure as sophisticated as the one we have here. Fortunately, the BEAR team helped me debug one important issue I had due to the setup of the BlueBEAR cluster being different from others I have worked on in the past. They also helped finding extra storage space so this computation was possible in the first place.
Whenever people ask me about my experience at the University of Birmingham, I always emphasise BEAR. I have never encountered a computer cluster, storage system, and infrastructure that operate as smoothly and reliably as they do here. It feels magical even though I know that this exceptional resource only functions so seamlessly on my end due to the hard work and dedication of the BEAR team. So kudos to you!
It feels magical even though I know that this exceptional resource only functions so seamlessly on my end due to the hard work and dedication of the BEAR team
I am now leveraging my detailed grid of stellar models to train a neural network. This neural network will enable me to implement a sophisticated Bayesian Hierarchical Model, allowing me to derive individual and population properties of groups of stars in a unified manner. While this approach presents its own challenges, it would have been nearly impossible to conduct this research without the comprehensive stellar grid computed on BlueBEAR and utilising the BEAR storage systems. These resources have been indispensable in enabling the advancement of my work.
We were so pleased to hear of how Amalie was able to make use of what is on offer from Advanced Research Computing, particularly to hear of how he has made use of BlueBEAR HPC and its many cores – if you have any examples of how it has helped your research then do get in contact with us at bearinfo@contacts.bham.ac.uk. We are always looking for good examples of use of High Performance Computing to nominate for HPC Wire Awards – see our recent winners for more details.