In this case study, we hear from Reza Omidyan (School of Chemistry), who has been utilising BEAR to predict the photophysical properties of biological and chemical compounds.
I am an associate professor of Physical Chemistry, and since a few months ago, I have been utilizing BlueBEAR, the supercomputer at the University of Birmingham for my research, in collaboration with Professor Vasilios Stavros (School of Chemistry).
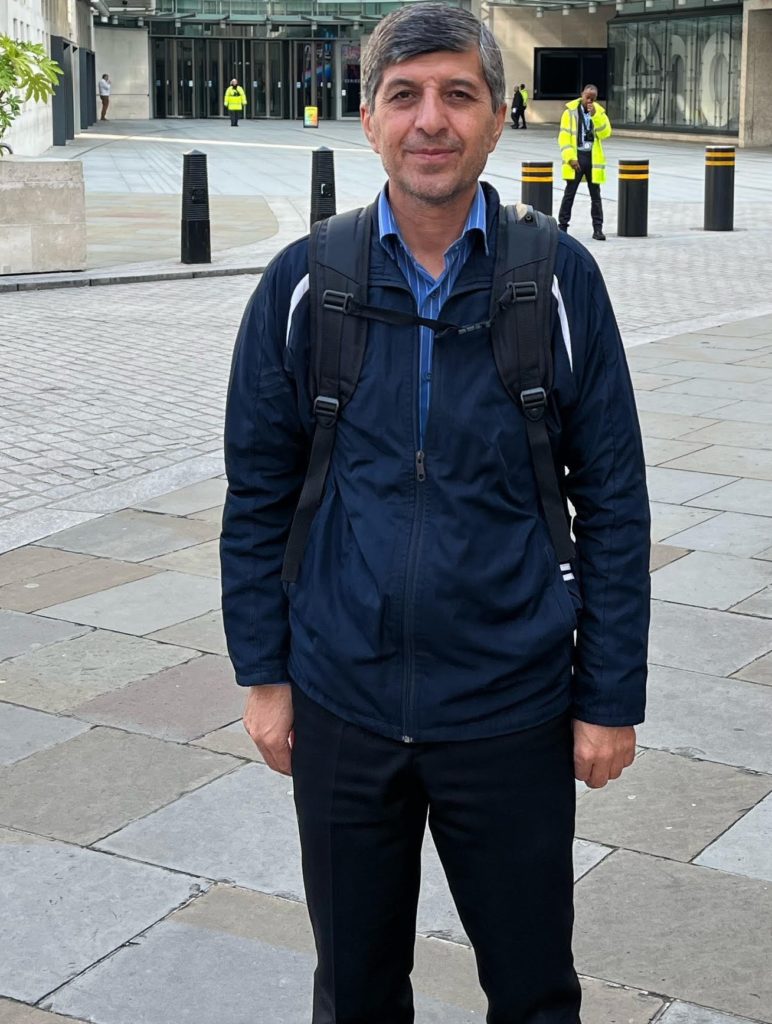
My work focuses on Molecular Photophysics, which involves studying the interaction of light with matter, aiming to predict the photophysical properties of biological and chemical compounds, including photostability, photoisomerization, and photoswitching. These properties are crucial for applications in health and material sciences, such as sunscreens and photostabilizers.
I am very grateful for the support provided by this impressive supercomputer facility, enabling us to look through large scale molecules and achieve nice results
To pursue my research, I employ high-level ab initio and nonadiabatic dynamics (NAD) simulation methods. Ab initio methods help determine optimized geometries, electronic structures, and potential energy surfaces. NAD simulations, which heavily rely on BlueBEAR, involve running hundreds of trajectories to track the evolution of excited state populations over time. These simulations address critical questions, such as the fraction of the population undergoing photoisomerization or relaxing to ground, and provide valuable insights into excited state relaxation mechanism.
Why BlueBEAR?
I typically study large size molecules. To analyse the dynamics of these systems, at least 100 trajectories must be executed over a timescale of 1000 femtoseconds (fs), with a time-step of 0.5 fs, each trajectory requires 2000 calculations of static quantum mechanical parameters (energy, gradient, etc.) and dynamic parameters (velocity, acceleration, etc., based on the Varlet algorithm). These calculations are extremely slow or even impossible on desktop computers, but BlueBEAR makes them feasible.
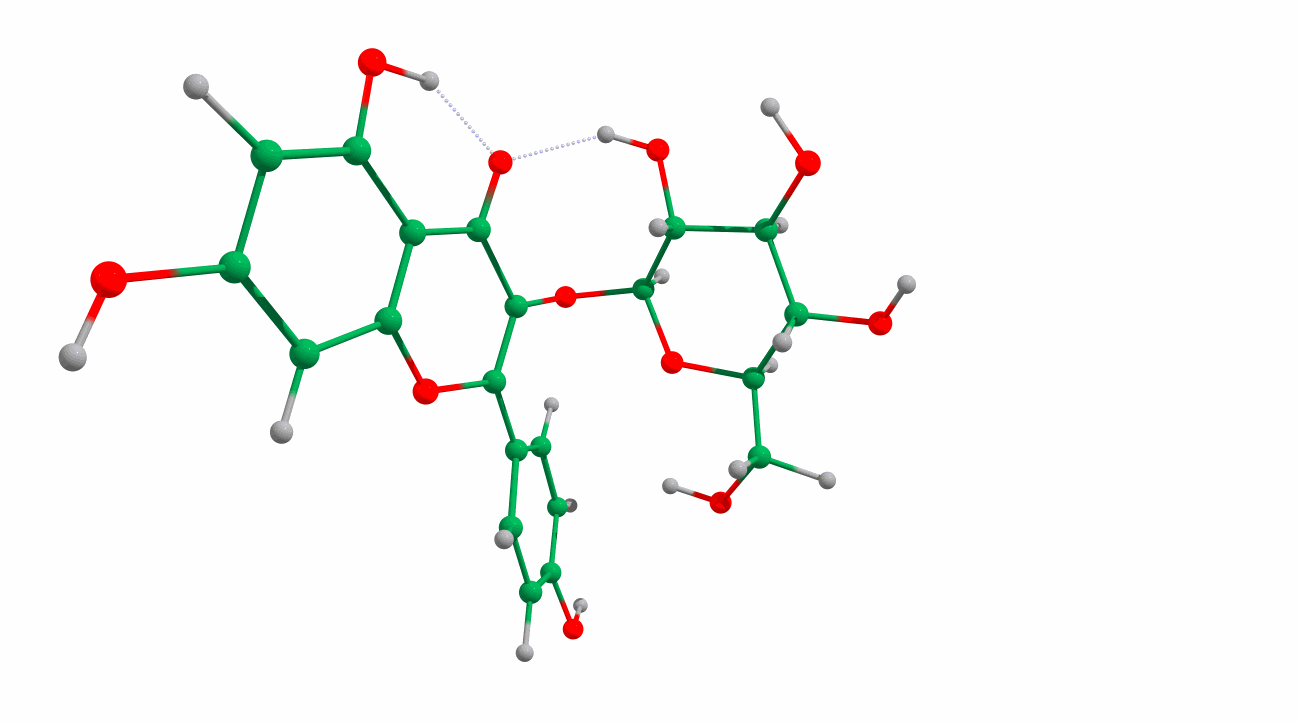
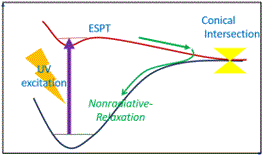
Figure 1: Left: animated single trajectory determined from the excited state evolution of Astragalin. Right: Deactivation mechanism proposed for this system.
In this project, the experimental group lead by Prof. Stavros, determine the Transient Absorption Electronic Spectroscopy (TEAS) spectra photodynamics, leading to the excited state lifetime. For Astragalin (Figure 1) this lifetime has been measured to be in the order of femtosecond. My NAD calculations on BlueBEAR unravelled that the deactivation mechanism is governed first by an excited state proton transfer (~50 fs), and then a ring deformation results in ultrafast internal conversion (IC), based on the S1/S0 conical intersection (CI).
I am very grateful for the support provided by this impressive supercomputer facility, enabling us to look through large scale molecules and achieve nice results.
We were so pleased to hear of how Reza was able to make use of what is on offer from Advanced Research Computing, particularly to hear of how he has made use of BlueBEAR HPC – if you have any examples of how it has helped your research then do get in contact with us at bearinfo@contacts.bham.ac.uk.
We are always looking for good examples of use of High Performance Computing to nominate for HPC Wire Awards – see our recent winner for more details.